Transistor
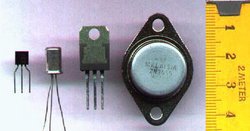
The transistor is a solid state semiconductor device which can be used for amplification, switching, voltage stabilization, signal modulation and many other functions. It acts as a variable valve which, based on its input current (BJT) or input voltage (FET), allows a precise amount of current to flow through it from the circuit's voltage supply.
In essence, a transistor has three terminals. A current or voltage applied through/across two terminals controls a larger current through the other terminal and the common terminal. In analog circuits, transistors are used in amplifiers. Analog circuits include audio amplifiers, stabilised power supplies and radio frequency amplifiers. In digital circuits, transistors function essentially as electrical switches. Digital circuits include logic gates, RAM (random access memory) and microprocessors.
Transistor was also the common name in the sixties for a transistor radio, a portable radio that used transistors (rather than vacuum tubes) as its active electronic components. This is still one of the dictionary definitions of transistor.
Invention
The transistor was invented at Bell Telephone Laboratories in December 1947 (first demonstrated on December 23) by John Bardeen, Walter Houser Brattain, and William Bradford Shockley, who were awarded the Nobel Prize in physics in 1956. Ironically, they had set out to manufacture a field-effect transistor (FET) predicted by Julius Edgar Lilienfeld as early as 1925 but eventually discovered current amplification in the point-contact transistor that subsequently evolved to become the bipolar junction transistor (BJT).
Origin of name
Bell Telephone Laboratories needed a generic name for their new invention: "Semiconductor Triode", "Solid Triode", "Surface States Triode" [sic], "Crystal Triode" and "Iotatron" were all considered, but "transistor", coined by John R. Pierce, won an internal ballot. The rationale for the name is described in the following extract from the company's Technical Memoranda (May 28, 1948) [1] calling for votes:
"Transistor. This is an abbreviated combination of the words "transconductance" or "transfer", and "varistor". The device logically belongs in the varistor family, and has the transconductance or transfer impedance of a device having gain, so that this combination is descriptive."
Importance
The transistor, considered by many to be one of the greatest inventions in modern history, ranks with the printing press and the telephone. It is the key active component in practically all modern electronics. Its importance in today's society rests on its ability to be mass produced using a highly automated process (fabrication) that achieves vanishingly low per-transistor costs.
Although millions of individual (discrete) transistors are still used, the vast majority are fabricated in chips along with diodes, resistors, capacitors and inductors to produce complete electronic functions, either analog or digital. Often both types of function are integrated on the same chip. The cost of designing and developing a complex chip is astronomical, but when spread across millions of production units the individual chip cost is minimized. A logic gate comprises about 20 transistors whereas an advanced processor, as of early 2005, can use as many as 289 million transistors.
The word "chip" is now used rather loosely: originally it referred to the actual piece of semiconductor before packaging. Once the chip had been packaged it was called an "integrated circuit", or just "IC", and sometimes a "bug". Chip, integrated circuit and IC are now used interchangeably while bug has gone out of fashion. The term "solid state" is used to describe a device which does not control charge flow through a vacuum (vacuum tube) or a gas and which does not use moving parts (relay) to control charge flow. In the same vein, a circuit or item of equipment may be described as "solid state".
The transistor's low cost, flexibility and reliability have made it an almost universal device for non-mechanical tasks. Whereas a common item, say a refrigerator, would have used electromechanical devices for control, today it is often less expensive and more effective to simply use a standard integrated circuit (containing a few million transistors) and write a computer program to carry out the same task through logic. Transistors have replaced almost all electromechanical devices, are used in most simple feedback control systems, and appear in huge numbers in everything from traffic lights to washing machines.
Hand-in-hand with low cost has come the trend to digitize information. With transistor-utilizing computers offering the ability to quickly find, sort and process digital information, more and more effort has been put into making information digital. Almost all media today is delivered in digital form, finally being converted and presented in analog form by computers. Familiar areas influenced by the drive to digitize are television, radio and newspapers.
Types
![]() | PNP | ![]() | P-channel |
![]() | NPN | ![]() | N-channel |
BJT | JFET |
Broadly speaking, transistors are categorised as follows:
- Semiconductor material: germanium, silicon, gallium arsenide
- Physical packaging: through hole metal, through hole plastic, surface mount, ball grid array
- Type: BJT, JFET, IGFET (MOSFET), "other types"
- Polarity: NPN/ N channel, PNP/ P channel
- Maximum power rating: low, medium, high
- Maximum frequency capability: low, medium, high, radio frequency (RF), microwave
- Application: amplifier, switch, general purpose, audio, high voltage
Thus, a particular transistor may be described as: silicon, surface mount, BJT, NPN, low power, high frequency switch.
FET Families
FETs are divided into two families: junction FET (JFET) and insulated gate FET (IGFET) also known as metal oxide silicon (or semiconductor) FET (MOSFET). Unlike IGFETs, the JFET gate terminal forms a diode with the channel (semiconductor material between source and drain). Functionally, this makes the N channel JFET the solid state equivalent of the vacuum tube triode which, similarly, forms a diode between its grid and cathode. Also, both devices operate in the "depletion mode", they both have a high input impedance, and they both conduct current under the control of an input voltage.
FETs are further divided into enhancement mode and depletion mode types. Mode refers to the polarity of the gate voltage with respect to the source when the device is conducting. Taking N channel FETs: in depletion mode the gate is negative with respect to the source while in enhancement mode the gate is positive. For both modes, if the gate voltage is made more positive the source/drain current will increase. For P channel devices the polarities are reversed. Most IGFETs are enhancement mode types and nearly all JFETS are depletion mode types.
Other types
- Unijunction transistors can be used as simple pulse generators. They comprise a main body of either P or N type semiconductor with ohmic contacts at each end (terminals B1 and B2). A junction with the opposite semiconductor type is formed at a point along the length of the body for the third terminal (E).
- Dual gate FETs have a single channel with two gates in cascode; a configuration that is optimised for high frequency amplifiers, mixers, and oscillators.
- Transistor arrays are used for general purpose applications, function generation and low-level, low-noise amplifiers. They include two or more transistors on a common substrate to ensure close parameter matching and thermal tracking, characteristics that are especially important for long tailed pair amplifiers.
- Darlington transistors comprise a medium power BJT connected to a power BJT. This provides a high current gain equal to the product of the current gains of the two transistors. Power diodes are often connected between certain terminals depending on specific use.
- Insulated gate bipolar transistors (IGBTs) use a medium power IGFET, similarly connected to a power BJT, to give a high input impedance. Power diodes are often connected between certain terminals depending on specific use. IGBTs are particularly suitable for heavy-duty industrial applications. The Asea Brown Boveri (ABB) 5SNA2400E170100 [2] illustrates just how far power semiconductor technology has advanced. Intended for three-phase power supplies, this awesome device houses three NPN IGBTs in a case measuring 38 by 140 by 190 mm. Each IGBT is rated at 1,700 volts and can handle 2,400 amperes.
Semiconductor material
Initially, BJTs were made from germanium (Ge) and some high power types still are. Silicon (Si) types currently predominate but certain advanced microwave and high performance versions now employ the compound semiconductor material gallium arsenide (GaAs). Single element semiconductor material (Ge and Si) is described as elemental. The characteristics for the most common semiconductor materials used to make transistors are given in the table below:
Semiconductor material | Junction
forward voltage V @ 25 °C | Electron mobility m/s @ 25 °C | Hole
mobility m/s @ 25 °C | Max junction temp. °C |
---|---|---|---|---|
Ge | 0.27 | 0.39 | 0.19 | 70 to 100 |
Si | 0.71 | 0.14 | 0.05 | 150 to 200 |
GaAs | 1.03 | 0.85 | 0.05 | 150 to 200 |
Al/Si junction | 0.3 | - | - | 150 to 200 |
The
Junction forward voltage is the voltage necessary to make the emitter/base
junction of a BJT conduct a specified current. The values given in the table are
typical for a current of 1 mA (the same values apply to semiconductor diodes).
The lower the junction forward voltage the better, as this means that less power
is required to "drive" the transistor. For normal doping levels the junction forward
voltage is typically 0.4 V less than the band gap. Band
gap is the voltage difference between the valence energy band and the conduction
energy band for a given material. The junction forward voltage for a given
current decreases with temperature. For a typical silicon junction the change
is around - 2 mV/°C.
Electron mobility and Hole mobility columns show the average speed that electrons and holes defuse through the semiconductor material with 1 volt per meter applied across the material. In general, the higher the electron mobility the faster the transistor. The table indicates that Ge is a better material than Si in this respect, and so it is. But, Ge has four major shortcomings compared to silicon and gallium arsenide: its maximum temperature is limited, it has relatively high leakage current, it can not withstand such high voltages and it is less suitable for fabricating chips. As the electron mobility is higher than the hole mobility for all semiconductor materials, a given NPN BJT tends to be faster than an equivalent PNP BJT type. GaAs has the fastest electron mobility of the three semiconductors. It is for this reason that GaAs is used in high frequency applications. A relatively recent FET development, the high electron mobility transistor (HEMT), has a hetro-structure (junction between different semiconductor materials) of aluminium gallium arsenide (AlGaAs)/gallium arsenide (GaAs) which has double the electron mobility of a GaAs/metal barrier junction. Because of their high speed and low noise, HEMTs are used in satellite receivers working at a frequency around 12 GHz.
Max junction temperature values represent a cross section taken from various manufacturer's data sheets. This temperature should not be exceeded or the transistor may be destroyed.
Al/Si junction refers to the aluminium/silicon high-speed semiconductor/metal barrier diode, commonly known as a schottky diode. This is included in the table because most silicon power IGFETs have a parasitic reverse schottky diode formed between the source and drain as part of the fabrication process.
Packaging
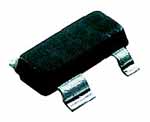
Transistors come in many different packages (see images), both through hole (or leaded) and surface mount, also known as surface mount device (SMD). Often you have a choice of package for a given transistor chip. The ball grid array (BGA) is the leading-edge surface mount package (currently only for large transistor arrays and digital functions). It has solder 'balls' on the underside which are fused to pads on the host printed circuit board (PCB) by heating the whole board, normally by infrared radiation. Being hidden, the joint is then checked for quality by x-ray imaging. Because they are smaller and have shorter interconnections, SMDs have better frequency characteristics but lower power rating. Transistor packages are normally made of glass, metal, ceramic or plastic. For enhanced cooling, power transistors have large packages that can be clamped to heat sinks, but at the other extreme some surface-mount microwave transistors resemble specks of dust. Also to aid cooling, most power types have the collector/drain internally connected to the metal can/metal plate (in "non-conductive" power packages the non-conductive package material is moulded on to a metal plate for heatsink interfacing).
Gallery
A bewildering range of transistors is already available and manufacturers continually introduce improved types. A few examples from the main families are featured below. Unless otherwise stated, all types are made from silicon semiconductor. Complementary pairs are shown as NPN/PNP or N/P channel. Just left click on the link[3] to access the data sheet (on some data sheets the stated transistor category can be misleading) :
- 2N3904[4]/2N3906[5], BC182[6]/BC212[7] and BC546[8]/BC556[9]. BJT, general-purpose, low-power, complementary pairs. They have plastic cases and cost a few cents U.S, making them popular with hobbyists.
- BFP183[10] Low power, 8 GHz microwave NPN BJT. High-frequency design techniques are mandatory if you want to make amplifiers, mixers and oscillators with Infineon's lightning-fast BJT. It costs around $1.0 U.S. WARNING: this device may contain beryllium oxide which is highly toxic: NEVER cut open or incinerate.
- LM394[11] 'supermatch pair' Two individual low power, low noise, NPN BJTs on a single substrate.
- 2N2219A[12]/2N2905A[13] BJT, general purpose, medium power, complementary pair. With metal cases they are rated at about one watt.
- 2N3055[14]/MJ2955[15] For years, the venerable NPN 2N3055 has been the standard power work-horse. Its complement, the PNP MJ2955 arrived later. These rugged, 1 MHz, 15 A, 60 V, 115 W BJTs are suitable for audio power amplifiers, power supplies and control. With metal or plastic cases they retail for about $1.00 U.S.
- 2SC3281/2SA1302 Optimised for the output stages of HiFi amplifiers up to 100 watts, Toshiba's low-distortion fully complementary, plastic case BJTs are revered by audiophile designers who are wary of botched counterfeits[16].
- BU508[17] NPN, 1,500 V, power BJT. Designed for television (TV) horizontal deflection, its high voltage capability also makes it useful for ignition systems.
- MJ11012[18]/MJ11015[19] 30 A, 120 V, 200 W, high power darlington complementary pair BJTs for audio amplifiers, control and power switching (high current reverse diode between emitter and collector).
- BSP296[22]/BSP171[23] IGFET (enhancement mode), medium power, near complementary pair (medium current reverse schottkey diode between source/drain). Infineon's ultra sensitive gate IGFETs are ideal for converting logic levels to higher voltages. They are also suitable for driving power transistors in amplifiers.
- IRF3710[24]/IRF5210[25] IGFET (enhancement mode), high power, near complementary pair (hign current reverse schottkey diode between source/drain). With remarkably low on-resistances of 23/60 milliohms and sensitive gates (10 V to fully turn on), International Rectifier's 40 A, 100 V, 200 W "heavy lifters" make efficient amplifiers and power switches, especially for automobiles.
Advantages of transistors over vacuum tubes
Before the development of transistors vacuum tubes (or in the UK thermionic valves or just valves) were the main active components in electronic equipment. The key advantages that have allowed transistors to replace their vacuum tube predecessors in most applications are:
- Smaller size (despite continuing miniaturization of vacuum tubes)
- Highly automated manufacture
- Lower cost (in volume production)
- Lower possible operating voltages
- Operate "immediately" (most vacuum tubes need 10 to 60 seconds to "warm up")
- Lower power dissipation (no heater power, very low saturation voltage)
- Higher reliability and greater endurance (but vacuum tubes are more resistant to nuclear electromagnetic pulses (NEMP) and electrostatic discharge (ESD) )
- Much longer possible lifetime (vacuum tube cathodes are eventually exhausted)
- Complementary devices available (allowing circuits with complementary symmetry: complementary vacuum tubes are not available)
- Ability to control large currents (power transistors are available to control hundreds of amperes: vacuum tubes to control even one ampere are large and costly)
- Non-microphonic (vibration can modulate vacuum tube characteristics)
"
Nature abhors a vacuum tube " John R. Pierce, Bell Telephone
Laboratories, circa 1948.
Use in audio amplifiers
From mobile phones to televisions, vast numbers of products include audio power amplifiers. The first discrete transistor types struggled to generate a few hundred milliwatts, but power and audio fidelity gradually increased as better transistors became available and amplifier architecture evolved (single-ended transformer coupled to push-pull transformer coupled to push-pull capacitor coupled to push-pull direct coupled).
In all but the most esoteric applications, chip audio power amplifiers have completely ousted discrete types. Initially these chips were temperamental and they too, could only deliver a few hundred milliwatts. But today, for around $10 U.S, you can make a pretty good 50 watt audio power amplifier with a few passive components and an LM3886[26] chip for example.
HiFi amplifiers
Some high fidelity (HiFi) audio amplifiers still use vacuum tubes with their enthusiasts claiming that their sound is superior.
In particular, some argue that the larger number of electrons in a vacuum tube behave with greater statistical accuracy, although this ignores the facts that vacuum tubes generally have a high-impedance control terminal (grid), and that discrete-transistor (as opposed to, say, op-amp) circuits can also be designed to use large currents (to put this in perspective, even 1 milliampere of current carries about 6,240 million million electrons per second).
Others detect a distinctive "warmth" to the sound. The "warmth" is actually distortion caused by the vacuum tubes, but some audiophiles find this pleasing. This is "soft-saturation" and occurs when vacuum tubes are overdriven, causing poorly designed vacuum tube amplifiers to sound better than poorly designed transistor amplifiers. Tube amplifiers are also less prone to slew-rate limiting, which was a problem with early semiconductors and is still observed in low-cost transistor audio amplifiers.
Above speculative opinion gives no correlation between characteristics or measurements and perceived sound quality. See Tubes vs. Transistors - Is There An Audible Difference? and Transistors vs. Tubes - Brief Feature Comparison.
Guitar amplifiers
Vacuum tubes are also preferred in guitar amplifiers which are designed to be overdriven, because they have a different non-linear transfer characteristic than transistors, and create a different, more pleasing spectrum of harmonic distortion or "fuzz". Digital signal processing (DSP) can be used to achieve similar effects in the digital domain.
It is possible to mix transistors and vacuum tubes in the same circuit.
Simple transistor amplifiers use emitter degeneration to achieve negative feedback, which gives a relatively predictable gain compared to the gain of the transistor itself, which varies widely.
Use in computers
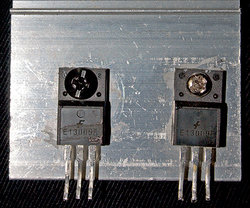
The "second generation" of computers through the late 1950s and 1960s featured boards filled with individual transistors, and magnetic cores. Subsequently, transistors, other components (capacitors, but not high-value inductors or transformers), and the necessary wiring were integrated into a single, mass-manufactured component: the integrated circuit. In modern digital electronics, single transistors are very rare, though they remain common in power and analog applications. Recently, inroads have been made in the integration of analog circuits, also, with the advent of "programmable analog" circuits, Systems-on-Chips, and Digital signal processing. DSP is a technique that can (among other things) be used with A/D and D/A converters to simulate analog circuits. Linear integrated circuits got a bad reputation early on because of the difficulty of creating (high-quality) PNP transistors, but are much better today.
Transistors are also used in power regulation and in computer PSUs, especially in switching power supplies.
How transistors work
There are two basic types of transistors, the bipolar junction transistor (BJT) and the field-effect transistor (FET), which work differently. Bipolar transistors are so named because the main conduction channel uses both electrons and holes to carry the main electric current. In BJTs the main current must cross a very thin insulating layer called the Depletion zone, and the width of this layer can be electrically varied at very high rates in order to control the main current. Field-effect transistors (also called unipolar transistors) use only one of the two types of carrier (either electrons or holes, depending on the subtype of the FET). In FETs the main current appears in a narrow conducting channel with an insulating Depletion zone at the side, and the width of this insulating zone can be altered by varying its applied voltage, in order to control the width of the conducting channel and therefore control the main current. See the articles on each type of device for more information.
How semiconductors work
Operationally, transistors and vacuum tubes have similar functions; they both control the flow of current.
In order to understand how a semiconductor operates, consider a glass container filled with pure water. If a pair of conductive probes are immersed in the water and a DC voltage (below the electrolysis point i.e. breakdown point for water) is applied between them, no current would flow because the water has no charge carriers. Thus, pure water is an insulator. Dissolve a pinch of table salt in the water and conduction begins, because mobile carriers (ions) have been released. Increasing the salt concentration increases the conduction, but not very much. A dry lump of salt is non-conductive, because the charge carriers are immobile.
An absolutely pure silicon crystal is also an insulator, but when an impurity e.g. arsenic is added (called doping) in quantities minute enough not to completely disrupt the regularity of the crystal lattice, it donates free electrons and enables conduction. This is because arsenic atoms have five electrons in their outer shells while silicon atoms have only four. Conduction is possible because a mobile carrier of charge has been introduced, in this case creating n-type silicon ("n" for negative. The electron has a negative charge).
Alternatively, silicon can be doped with boron to make p-type silicon which also conducts. Because boron has only three electrons in its outer shell another kind of charge carrier, called a "hole", is formed in the silicon crystal lattice.
In a vacuum tube, on the other hand, the charge carriers (electrons) are emitted by thermionic emission from a cathode heated by a wire filament. Therefore, vacuum tubes cannot generate holes (positive charge carriers).
Note that charge carriers of the same polarity repel one another so that, in the absence of any force, they are distributed evenly throughout the semiconductor material. However, in an unpowered bipolar transistor (or junction diode) the charge carriers tend to migrate towards a P/N junction, being attracted by their opposite charge carriers on the other side of the junction.
Increasing the doping level increases the semiconductor conductivity, providing that the crystal lattice, overall, remains intact. In a bipolar transistor the emitter has a higher doping level than the base. The ratio of emitter/base doping levels is one of the main factors that dictates the junction transistor's current gain.
The level of doping is extremely low: in the order of parts per one hundred million, and this is the key to semiconductor operation. In metals, the carrier population is extremely high; one charge-carrier per atom. In metals, in order to convert a significant volume of the material into an insulator, the charge carriers must be swept out by applying a voltage. In metals this value of voltage is astronomical; more than enough to destroy the metal before it converts to an insulator. But in lightly-doped semiconductors there is only one mobile charge carrier per millions or more atoms. The level of voltage required to sweep so few charge-carriers out of a significant volume of the material is easily reached. In other words, the electricity in metals is incompressible, like a fluid, while in semiconductors behaves as a compressible gas. Doped semiconductors can be rapidly changed into insulators, while metals cannot.
The above explains conduction in a semiconductor by charge carriers, either electrons or holes, but the essence of bipolar transistor action is the way that electrons/holes seemingly make a prohibited leap across the insulating depletion zone in the reverse-biased base/collector junction under control of the base/emitter voltage. Although a transistor may seem like two interconnected diodes, a bipolar transistor cannot be made simply by connecting two discrete junction diodes together. To produce bipolar transistor action they need to be fabricated on the same crystal, and physically sharing a common and extremely thin base region.
Electrical characteristics
Note that in the following example I am using the convention that current flows "from" the positive "to" the negative. In actuality, the moving charge carrier, the electron, is negatively charged and moves in the opposite direction.
When analyzing how current will flow through a circuit, a bipolar transistor can be thought of as effectively three diodes, two of which always conduct, and one of which will conduct depending on the current flowing through the other two. In an NPN transistor, for example, the base will conduct to either the collector or emitter without any resistance other than a fixed forward voltage drop, so long as the voltage at the base is higher than at the emitter or collector. In addition, if current is flowing through the base-emitter junction, a much larger current will be allowed to flow from the collector to the emitter. The multiple that the allowed emitter-collector current is larger than the base-emitter current is called gain or hFE. An hFE value of 100 is typical for small bipolar transistors.
In a typical configuration, a very small signal current flows through the base-emitter junction to control the emitter-collector current. However, bipolar transistors are approximately symmetrical (sans doping differences), and you can use a base-collector current to control the emitter-collector current. Generally the gain in this mode is much smaller (i.e., 2 instead of 100), and it is not a value that is controlled by manufacturers so it can vary wildly.
Light sensitivity
Bipolar transistors can be turned on with light as well as electricity. Devices designed for this purpose are called phototransistors, but these can be standard transistors in a transparent package.
History
All transistors rely on the ability of certain materials, known as semiconductors, to change their conduction under the control of an electric field. In bipolar transistors, the semiconductor is formed into structures called p-n junctions that allow electricity to flow in only one direction through them – that is, they are a conductor when voltage is applied in one direction, and an insulator when it is applied in the other direction.
1900s
Semiconductors had been used in the electronics field for some time before the invention of the transistor. Around the turn of the 20th century they were quite common as detectors in radios, used in a device called a "cat's whisker". These detectors were somewhat troublesome, however, requiring the operator to move a small tungsten filament (the whisker) around the surface of a crystal until it suddenly started working. Then, over a period of a few hours or days, the crystal would slowly stop working and the process would have to be repeated. At the time their operation was completely mysterious. After the introduction of the more reliable and amplified vacuum tube based radios, the cat's whisker systems quickly disappeared. The "cat's whisker" is a primitive example of a special type of diode still popular today, called a Schottky diode.
World War II
In WWII, radar research quickly pushed the frequencies of the radio receivers inside them into the area where traditional tube based radio receivers no longer worked well. On a whim, Russell Ohl of Bell Laboratories decided to try a cat's whisker. After hunting one down at a used radio store in Manhattan, he found that it worked much better than tube-based systems.
Ohl investigated why the cat's whisker functioned so well. He spent most of 1939 trying to grow more pure versions of the crystals. He soon found that with higher quality crystals the "oddness" went away, but so did their ability to operate as a radio detector. One day he found one of his purest crystals nevertheless worked well, and interestingly, it had a clearly visible crack near the middle. However as he moved about the room trying to test it, the detector would mysteriously work, and then stop again. After some study he found that the behaviour was controlled by the light in the room – more light, more conductance. He invited several other people to see this crystal, and Brattain immediately realized there was some sort of junction at the crack.
Further research cleared up the remaining mystery. The crystal had cracked because either side contained very slightly different amounts of the impurities Ohl could not remove – about 0.2%. One side of the crystal had impurities that added extra electrons (the carriers of electrical current) and made it a conductor. The other had impurities that wanted to bind to these electrons, making it an insulator. When the two were placed side by side the electrons could be pushed out of the side with extra electrons (soon to be known as the emitter) and replaced by new ones being provided (say from a battery) where they would flow into the insulating portion and be collected by the filament (the collector). However, when the voltage was reversed the electrons being pushed into the collector would quickly fill up the "holes", and conduction would stop almost instantly. This junction of the two crystals (or parts of one crystal) created a solid-state diode, and the concept soon became known as semiconduction. 'Anode' and 'Cathode' are the terms used to denote the two terminals of a diode. The mechanism of action when the diode is off has to do with the separation of charge carriers around the junction. This is called a 'depletion region.'
Development
Armed with the knowledge of how these new diodes worked, a crash effort started to learn how to build them on demand. Teams at Purdue University, Bell Labs, MIT, and the University of Chicago all joined forces to build better crystals. Within a year germanium production had been perfected to the point where military-grade diodes were being used in most radar sets.
The key to the development of the transistor was the further understanding of the process of the electron mobility in a semiconductor. It was realized that if there was some way to control the flow of the electrons from the emitter to the collector, one could build an amplifier. For instance, if you placed contacts on either side of a single type of crystal the current would not flow through it. However if a third contact could then "inject" electrons or holes into the material, the current would flow.
Actually doing this appeared to be very difficult. If the crystal were of any reasonable size, the amount of electrons (or holes) supplied would have to be very large — making it less than useful as an amplifier because it would require a large current to start with. That said, the whole idea of the crystal diode was that the crystal itself could provide the electrons over a very small distance. The key appeared to be to place the input and output contacts very close together on the surface of the crystal.
Brattain started working on building such a device, and tantalizing hints of amplification continued to appear as the team worked on the problem. One day the system would work and the next it wouldn't. In one instance a non-working system started working when placed in water. The two eventually developed a new branch of quantum mechanics known as surface physics to account for the behaviour.
Essentially, the electrons in any one piece of the crystal would migrate about due to nearby charges. Electrons in the emitters, or the "holes" in the collectors, would cluster at the surface of the crystal where they could find their opposite charge "floating around" in the air (or water). Yet they could be pushed away from the surface from any other location with the application of a small amount of charge. So instead of needing a large supply of electrons, a very small number in the right place would do the trick.
Their understanding solved the problem of needing a very small control area to some degree. Instead of needing two separate semiconductors connected by a common, but tiny, region, a single larger surface would serve. The emitter and collector would both be placed very close together on one side, with the control lead on the other. When current was applied to the control lead, the electrons or holes would be pushed out, right across the entire block of semiconductor, and collect on the far surface. As long as the emitter and collector were very close together, this should allow enough electrons or holes between them to allow conduction to start.
First transistor
They made many attempts to build such a system with various tools, but generally failed. Setups where the contacts were close enough were invariably as fragile as the original cat's whisker detectors had been, and would work briefly, if at all.
Eventually they had a practical breakthrough. A piece of gold foil was glued to the edge of a plastic wedge, and then the foil was sliced with a razor at the tip of the triangle. The result was two very closely spaced contacts of gold. When the plastic was pushed down onto the surface of a crystal and voltage applied to the other side (on the base of the crystal), current started to flow from one contact to the other as the base voltage pushed the electrons away from the base towards the other side near the contacts. The point-contact transistor had been invented, a primitive variation of the BJT.
While the device was constructed a week earlier, Brattain's notes describe the first demonstration to higher-ups at Bell Labs on the afternoon of December 23, 1947, often given as the birthdate of the transistor. The PNP point-contact germanium transistor operated as a speech amplifier with a power gain of 18 in that trial.
Problems with fragility and impurities
Shockley was upset about the device being credited to Brattain and Bardeen, who he felt had built it "behind his back" to take the glory. Matters became worse when Bell Labs lawyers found that some of Shockley's own writings on the transistor were close enough to those of an earlier patent that they thought it best that his name be left off the patent application.
Shockley was incensed, and decided to demonstrate who was the brains of the operation. Only a few months later he invented an entirely new type of transistor one day while sitting in his hotel room waiting to give a speech. This new form, the layer transistor, was considerably more robust than the fragile point-contact system, and would go on to be used for the vast majority of all transistors into the 1960s.
With the fragility problems solved, a remaining problem was purity. Making germanium of the required purity was proving to be a serious problem, and limited the number of transistors that actually worked from a given batch of material. One then-small company, Texas Instruments, decided that the solution to this problem was to use silicon rather than germanium, which should be easier to work with. They were right, and germanium disappeared from most transistors by the late 1960s.
Now everything was in place, and within a few years, transistor-based products, most notably radios, were appearing on the market. A major improvement in yield came when a chemist advised the companies fabricating semiconductors to use distilled water rather than tap water: calcium ions were the cause of the problem. 'Zone melting', also known as 'zone refining', a technique using a moving band of molten material through the crystal, makes this whole endeavour possible.
Early consumer and hobbyist applications
Contrary to popular belief, the portable radio was not the first 'mainstream' transistor application. Even in the 1940s, consumer radios were rather sophisticated; with several vacuum tubes they used Armstrong's brilliantly ingenious superheterodyne architecture. To meet the public's expectations, it would have been necessary for transistor radios to use similar circuitry but early transistors could not operate as amplifiers and oscillators at radio frequencies, even the relatively low 540 to 1700 "kilocycle" AM broadcast band. Also, miniature passive components, such as intermediate frequency transformers and multigang tuning capacitors, were not readily available.
Instead, the first major consumer application was the hearing aid, which only required audio amplification, and retailed in a market where miniaturization was important but low cost not essential. Raytheon, who had also developed ruggedized, minature vacuum tubes for the military, introduced the first transistorized hearing aid.
Texas Instruments' Regency TR-1 [27], the first transistor radio for the general public, wasn't marketed until 1954. But transistor radio sales only really took-off in 1958 with the launch of Sony's model TR-55.
Costing $12 U.S, the PNP CK722 [28] hit the market in early 1953. It was practically the only transistor available to hobbyists for almost a decade and innumerable homebrew projects were built using it. The retail versions had failed quality control (QC) and were not suitable for Raytheon's new hearing aid. They had low current gain, high leakage current, especially at elevated temperature, and the characteristics varied between individual parts. They were also a bit fragile and easily "blown up". This made designing practical circuits with the CK722 challenging. In spite of these shortcomings, many hobbyists of the fifties still have a warm place in their hearts for this early germanium transistor.
Miniaturization
With the introduction of transistors, electronic equipment shrank. Another leap in miniaturisation came when complete circuits were integrated on to tiny silicon chips.
The invention of metal oxide silicon (MOS) transistors by the Radio Corporation of America (RCA) in 1960, led to the development of PMOS and NMOS chips that integrated more functions, used less power and were cheaper, but were slower than BJT types. They followed this in 1967 by producing complementary metal oxide silicon CMOS chips that were faster than the previous MOS families and used even less power. With their high level of integration, low cost and minuscule power consumption MOS chips spawned a new generation of battery powered products such as pocket calculators and digital watches.
Advances in packaging have also shrunk components: currently the Ball Grid Array (BGA) package is the most compact. This was preceded by the standard surface mount device (SMD) packages for chips, transistors, diodes, transformers, inductors, capacitors and resistors.
Compared to the massive expenditure of launching items into space on-board liquid-fuelled rockets, the individual electronic component costs were insignificant. More important was the advantages of reliability and small size. This is why the National Aeronautics and Space Administration (NASA) could justify paying the equivalent of $4,000 U.S. in today's values, for an early quad two-input NAND gate. Now, an equivalent chip, but with vastly improved characteristics, would cost a few cents U.S. while the marginal cost of integrating an additional nand gate into a very large scale integration (VLSI) chip would be essentially zero.
See also
- Bipolar junction transistor
- Darlington transistor
- Semiconductor
- Integrated circuit vacuum tube
- NPN
- PNP
- Transconductance
- Transresistance
- Vacuum tube
- Band gap
External links and references
- U.S. Patent 2524035 -- J. Bardeen et. al.
- U.S. Patent 2569347 -- W. Shockley
- AudioUK's Milestones. Photograph of first working transistor
- Transistorized. Historical and technical information from the Public Broadcasting Service (PBS) web site
- The Transistor Legacy Then and Now. From Lucent Technologies (Bell Telephone Laboratories) (AT&T)
- This Month in Physics History: November 17 to December 23, 1947: Invention of the First Transistor. From the American Physical Society (APS)
- 50 Years of the Transistor. From Science Friday, December 12, 1997
- The CK722 Museum. Website devoted to the "classic" hobbyist germanium transistor
- Bob's Virtual Transistor Museum & History. Treasure trove of transistor history
- 1954 to 2004, the TR-1's Golden Anniversary. In depth coverage of Regency radio.
- Jerry Russell's Transistor Cross Reference Database.
- Transistor Tutorial.
- Riordan, Michael & Hoddeson, Lillian (1998). Crystal Fire. W.W Norton & Company Limited. ISBN 0393318516. The invention of the transistor & the birth of the information age
- Amos S W & James M R (1999). Principals of Transistor Circuits. Butterworth-Heinemann. ISBN 0750644273.
- Horowitz, Paul & Hill, Winfield (1989). The Art of Electronics. Cambridge University Press. ISBN 0521370957.
- Warnes, Lionel (1998). Analogue and Digital Electronics. Macmillan Press Ltd. ISBN 0-333-65820-5.
- Williams, Tim (2001). EMC for Product Designers (third edition). Butterworth Heinemann. ISBN 0-7506-4930-5.